LED indicator
In this encyclopedia, an LED indicator is defined as a component usually 10mm or smaller in diameter, made of transparent or translucent epoxy or silicone, most often containing one light-emitting diode. It is purposed as a status indicator in a device, rather than as a source of illumination, and is sometimes referred to as a standard LED.
LED indicators that emit infrared and ultraviolet light are included in this entry. LEDs that are designed to illuminate large interior or exterior areas are discussed in a separate entry as LED area lighting. They are sometimes described as high-brightness LEDs and almost always emit white light.
The term light-emitting diode is becoming less common, as the acronym LED has become ubiquitous. The acronym does not usually include periods between the letters.
The words “light emitting” are hyphenated here, as they form an adjectival phrase, but in everyday usage the hyphen is often omitted, and no definitive rule seems to exist.
Originally, a standard LED contained only one diode, but may now include multiple di- odes, either to emit additional light or to provide a range of colors. In this encyclopedia, a single epoxy or silicone capsule is still considered to be an LED indicator regardless of how many diodes it contains. By contrast, any component consisting of multiple sep- arately discernible light-emitting diodes, as in a seven-segment numeral, a 14- or 16- segment alphanumeric character, a dot-matrix character, or a display of multiple char- acters, is listed in a separate entry as an LED display.
What It Does
An LED indicator emits light in response to a small current, typically around 20mA (but some- times much less), at a voltage lower than 5VDC. It is usually molded from epoxy or silicone that may be colorless and transparent (often referred to as water clear), or colorless but translucent, or tinted and transparent, or tinted and translucent.
The color of the light is initially determined by the chemical compounds used internally, and by their dopants; therefore, a water-clear LED may emit colored light.
Ultraviolet LEDs are usually water-clear. Infrared LEDs often appear to be black, because they are opaque to the visible spectrum while being transparent to infrared.
When an LED indicator is described as being through hole, it has leads for insertion into holes in a circuit board. The term does not mean that the indicator itself is meant to be pushed through a hole in a panel, although this may also be done. The LED is cylindrical with a hemispherical top that acts as a lens. The leads are relatively thick, to conduct heat away from the component. A dimensioned diagram of a typical LED measuring 5mm in diameter is shown in Figure 22-1.
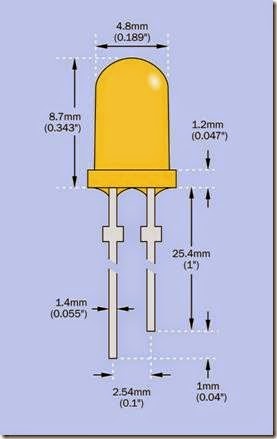
Figure 22-1. Dimensions of a typical 5mm LED. The longer wire connects with the anode, while the shorter wire connects with the cathode. Adapted from a data- sheet published by Lite-On Technology Corporation.
An LED indicator that is not the through-hole type is usually a surface-mount component. LEDs for surface mounting are mostly rectangular and can be as small as 1mm x 0.5mm. They may require a heat sink.
Schematic Symbols
Figure 22-2 shows a variety of symbols that are commonly used to represent an LED. The triangle at the center of each symbol points in the direction of conventional (positive-to-negative) cur- rent flow—from the anode to the cathode. Each pair of arrows radiating away from the diode indicates emitted light. Wavy arrows are some- times used to represent infrared (thermal) radiation. Often, however, an infrared LED is represented in exactly the same style as an LED that emits visible light. With the exception of the wavy arrows, the various styles of schematic symbol are functionally identical and do not identify different attributes of the component such as size or color.
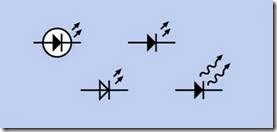
Figure 22-2. Various symbols may be used to represent an LED. See text for details.
Common Usage
LED indicators have mostly displaced neon bulbs and miniature incandescent lamps for the purpose of showing the status of a device. They are found in industrial control panels, home audio systems, battery chargers, washer/dryers, and many other consumer-electronics products. Higher output variants are used in flashlights, traffic signals, taillights on automobiles, and for illumination of subjects that are being photo- graphed. LED indicators may be assembled in large numbers in attention-getting displays such as Christmas lights.
Red, orange, yellow, green, and blue are the basic standard colors. LEDs that appear to generate white light are common, but they do not emit an evenly weighted spectrum of wavelengths. See “Non-Incandescent Sources” on page 174 for a discussion of this topic.
How It Works
An LED, like any diode, contains a semiconductor PN junction that conducts current only in the forward direction (i.e., from the more-positive side of a power supply to the more-negative side). The diode becomes conductive above a threshold voltage sufficient to force electrons in the n-type region and holes in the p-type region to combine with each other. Each time this oc- curs, energy is released. The energy liberated by one electron-hole combination creates a pho- ton, or one quantum of light.
The amount of energy released depends on the band gap, which is a property of the semiconductor material. The band gap is the smallest energy that can create an electron-hole pair. The energy determines the light’s wavelength, and thus the color.
The band gap also determines the threshold voltage of the LED. For this reason, LEDs of different colors have widely different threshold voltages.
Because an LED will often be used in devices where the DC power supply exceeds the maxi- mum forward voltage, a series resistor is customarily used as a simple way to restrict current through the diode.
The light emitted by a colored LED indicator tends to include only a narrow range of wave- lengths. However, the addition of a phosphor coating to the diode can broaden the output. This technique is used to make the light from a blue LED appear white, as shown in Figure 22-3. Most white LEDs are actually blue LEDs with a colored phosphor layer added. See the section
on LED area lighting in Chapter 23 for a more detailed discussion of this topic.
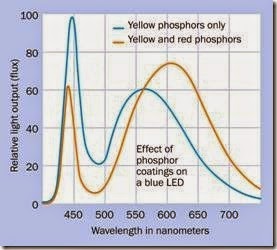
Figure 22-3. Increasing the range of emitted wavelengths by adding phosphors to a blue LED. Source: Philips Gard- co Lighting.
Multicolor LEDs and Color Mixing
If red, green, and blue light sources are mounted extremely close together, the eye perceives them as a single source, of a color determined by their combined relative intensities. This system of additive color mixing is shown in Figure 17-14 in the entry dealing with LCDs. It is used in LED indicators that contain red, green, and blue light- emitting diodes in a single epoxy or silicone capsule.
While most video monitors use white LEDs or fluorescent lights to form a backlight for an LCD video screen, some high-end monitors use a matrix of very tiny red, green, and blue LEDs, because the combination of these separate col- ors generates a wider gamut of color wave- lengths. The concept of gamut is discussed in “Color” in the LCD entry. The tiny LEDs in a back- light cannot be considered as indicators, but in- dicators are used for this purpose in billboard- sized video displays.
Variants
LED indicators vary widely in size, shape, intensity, view angle, diffusion of light, wavelength of light, minimum and maximum forward voltage, and minimum and maximum forward current.
Size and Shape
The original sizes for round LED indicators were 3mm, 5mm, or (more rarely) 10mm in diameter. Today, through-hole LEDs are sold in many inter- mediate sizes, although 3mm and 5mm are still most widely used.
The traditional round LED indicator is now augmented with square and rectangular shapes. In a parts catalog, a pair of dimensions such as 1mm × 5mm suggests that the LED is rectangular.
Intensity
The light intensity of an LED is usually expressed in millicandelas, abbreviated mcd. There are 1,000 mcd in a candela. For more information about units for measurement of light, see “Inten- sity” on page 178.
The candela measures the luminous flux, or visible radiant power, contained within a specified angle of dispersion, usually referred to as the view angle. This can be imagined as the rotated angle at the apex of a cone, where the cone de- fines the “spread” of the light, and the source is at the apex.
If a diode is emitting a fixed amount of luminous flux, the rating in mcd will increase with the in- verse square of the view angle. This is because the light delivered to an area in front of the LED will become more intense as the angle becomes smaller. The use of mcd to rate the brightness of an LED can be misleading if it is not considered in comparison with the view angle.
For example, suppose an LED is rated at 1,000 mcd and has a view angle of 20 degrees. Now suppose the same diode is embedded in a different epoxy or silicone capsule with a lens that creates a view angle of only 10 degrees. The LED
will now be rated at 4,000 mcd, even though its total power output is unchanged.
• To compare the brightness of two LED indicators meaningfully, they should share the same view angle.
Four through-hole LED indicators with a wide range of specifications are shown in Figure 22-4. From left to right: water-clear white generic, 10mm; Vishay TLCR5800 5mm (emitting red, even though the capsule is water-clear), rated for 35,000mcd with 4 degrees view angle; Everlight HLMPK150 5mm red diffused, rated for 2mcd with 60 degrees view angle; and Chicago 4302F5-5V 3mm green, rated for 8mcd at 60 de- grees view angle, containing its own series resistor to allow direct connection with a 5VDC power supply.
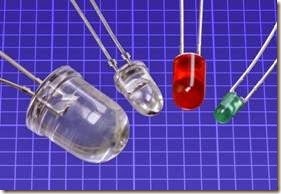
Figure 22-4. Four assorted LED indicators with very different specifications. See text for details.
Efficacy
The radiant luminous efficacy (LER) of an incandescent light source compares how effective it is at channeling its output within the visible spectrum, instead of wasting it in other wavelengths, especially infrared. Note that the word efficacy has a different meaning from efficiency. The LER acronym may help to avoid confusion.
LER is expressed in lumens per watt, and in an incandescent bulb it is calculated by dividing the power emitted in the visible spectrum (the luminous flux) by the power emitted over all wave- lengths. This is described in detail on “Efficacy” on page 179 in the entry dealing with incandescent lamps.
In an LED indicator, almost all the radiation can be within the visible spectrum, but some power is wasted by generating heat internally. The efficacy varies depending on the type of LED; thus a red-orange indicator can have an efficacy of 98% while a blue LED will be probably below 40%.
Diffusion
Some LED indicators use epoxy or silicone that is formulated to be translucent or “cloudy” instead of transparent. They diffuse the light so that it is not projected in a defined beam, has a softer look, and has an approximately equal intensity when viewed from a wider range of angles.
“Clear” and “diffused” are options that must be taken into account when choosing LEDs from an online catalog, unless the user is willing to turn a clear LED into a diffuse LED by applying some sandpaper.
Wavelength and Color Temperature The wavelength of light is measured in nanometers (abbreviated nm), a nanometer being 1 billionth of a meter. The visible spectrum extends
from approximately 380nm to 740nm. Longer wavelengths are at the red end of the spectrum, while shorter wavelengths are at the blue end.
A typical LED emits a very narrow range of wave- lengths. For example, Figure 22-5 shows the emission from a standard red LED indicator manufactured by Lite-On. Graphs of this type are typically included in manufacturers’ datasheets.
Because a red LED stimulates the cones in the eye that respond to red light, it “looks red” even though the color is not comparable with the natural red that is seen, for instance, in a sunset. That natural color actually contains an additional spread of wavelengths.
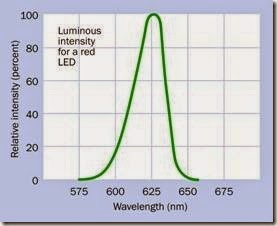
Figure 22-5. The narrow range of wavelengths emitted by a typical red LED indicator.
The following list shows the ranges of peak out- put values, in nanometers, for the most commonly available basic LED indicators (LEDs that emit other wavelengths are available, but they are less common):
• Infrared LED: 850 to 950
• Red LED: 621 to 700
• Orange LED: 605 to 620
• Amber LED: 590 to 591
• Yellow LED: 585 to 590
• Green LED: 527 to 570
• Blue LED: 470 to 475
• Ultraviolet LED: 385 to 405
Figure 22-6 shows this list graphically, omitting infrared and ultraviolet LEDs.
For almost 30 years, blue LEDs were a laboratory curiosity of little practical value, as efficiencies were stuck around 0.03%. An efficiency of more than 10% was finally achieved in 1995. Blue LEDs were marketed soon afterward.
However, when yellow phosphors are added to create the impression of white light by spreading the output over the whole visible spectrum, the wavelengths around 500nm are still not well rep- resented, as suggested in Figure 22-3.
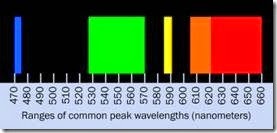
Figure 22-6. Ranges for peak wavelengths of the most commonly used LEDs. (Source: Survey of approximately 6,000 through-hole LEDs stocked at www.mouser.com.)
Fluorescent lights perform even more poorly than white LEDs, as can be seen in Figure 18-4 in the entry describing incandescent lamps.
Because white LEDs do not emit a single peak of wavelengths, their color is expressed in color temperature rather than nanometers. The concept of color temperature is explained in “Spectrum” on page 173. White LEDs are available rated from 2,800 to 9,000 degrees Kelvin, and are discussed in more detail in the LED area lighting entry in this encyclopedia.
Internal Resistor
To eliminate the chore of adding a series resistor to limit current through an LED, some indicators are sold with a series resistor built in. They may be rated for use with 5VDC or 12VDC, but are externally indistinguishable from each other. They are also externally indistinguishable from LEDs that do not contain series resistors. Figure 22-7 shows two 3mm LEDs, the one on the right containing its own series resistor, the one on the left being a generic LED without a series resistor.
Because of the nonlinear response of a diode, LEDs with or without internal resistors cannot be distinguished from each other reliably with a multimeter. If the meter is set to measure ohms, typically it will give an “out of range” error to all types of LED. If it is set to identify a diode, the reading will not tell you if the LED contains a resistor.
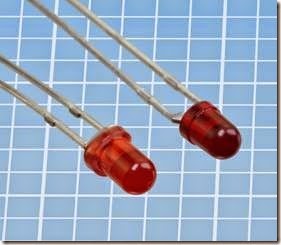
Figure 22-7. An LED (left) that does not contain its own series resistor is usually indistinguishable from one that does (right).
One way to determine whether an LED contains an internal series resistor would be to connect it with a variable power supply through a multi- meter set to measure mA. Carefully increase the supply voltage from zero until the meter shows a current of 20mA. If the LED does not contain a series resistor, the supply voltage will be close to the recommended forward voltage for that type of LED (no lower than 1.6V for a red LED, and no higher than 3.6V for a white LED). If the LED does contain a series resistor, the supply voltage will be higher. This procedure is time consuming, but may be worthwhile to evaluate multiple LEDs that are known to be identical.
Multicolored
The leads for an LED indicator containing two or more diodes can be configured in several ways:
• Two leads, two colors. Two diodes are mounted internally in parallel, but with opposite polarity.
• Three leads, two colors. Two diodes share a common anode or common cathode.
• Four leads, three colors (RGB). Three diodes share a common anode or common cathode.
• Six leads, three colors. Three diodes, each with its own pair of leads, separate from the others.
Infrared
Most infrared emitters are LEDs that generate wavelengths longer than 800nm. They are found in handheld remotes to control consumer- electronics devices such as televisions and stereo systems, and are also used in some security systems, although passive infrared motion detectors, which assess infrared radiation from sources such as people or vehicles, are more commonly used for this purpose.
In conjunction with an infrared emitter, an infra- red sensor is necessary, and must be sensitive to the same wavelength. To prevent false positives, the emitter modulates its output, typically with a carrier frequency between 10 and 100kHz. Remotes often use carrier frequencies of 30 to 56kHz. At the receiving end, the signal is processed with a band-pass filter matching the modulation frequency. Many different pulse-coding schemes are used, and no particular standard is dominant.
Ultraviolet
Because ultraviolet radiation can damage the eyes, LED indicators that emit ultraviolet light are potentially dangerous and should be used with caution. A yellow eyeshield can be worn to block the short wavelengths.
Ultraviolet light can be used to cure some adhesives and dental filling material. It can also kill bacteria, and can detect fluorescent print on bank notes, to check for counterfeiting. Ultraviolet flashlights are sold to detect some species of pests, such as scorpions, which fluoresce in re-sponse to ultraviolet light.
Values
The specification for an LED will include the wavelength of emitted light, luminous intensity, maximum forward voltage and current, maximum reverse voltage and current, and working values for voltage and current. All these values are important when choosing an indicator for a specific function.
White LEDs for room lighting or external use are calibrated differently. See the entry for LED area lighting in Chapter 23.
Forward Current
About half of all the thousands of available types of LED indicators are rated for a typical forward current of 20mA to 25mA. Absolute maximum ratings may be twice as high, but should not generally be applied.
The light intensity of a typical 5mm red LED indicator is plotted against its forward current in Figure 22-8. Note that current and light intensity have an approximately linear relationship up to the typical working current of 20mA. Even above this point, to the absolute maximum of 50mA, the light intensity rolls off only a very small amount.
Although an LED indicator can be dimmed by controlling the current passing through it, the current does not have a linear relationship with the applied voltage, and the indicator will stop functioning completely when the voltage drops below the threshold required by the diode. Consequently, LEDs are commonly dimmed by using pulse-width modulation.
Because of the nonlinear response of a diode, LEDs with or without internal resistors cannot be distinguished from each other reliably with a multimeter. If the meter is set to measure ohms, typically it will give an “out of range” error to all types of LED. If it is set to identify a diode, the reading will not tell you if the LED contains a resistor.
Low-Current LEDs
Indicators that require a very low forward current are convenient for direct connection to output pins of logic chips and other integrated circuits. Although a single output from an HC family chip is capable of supplying 20mA without damaging the chip, the current will pull down the output voltage, so that it cannot be used reliably as an input to another chip while also lighting the LED.
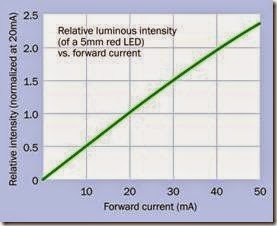
Figure 22-8. The relationship between forward current and light intensity of a typical 5mm LED indicator is approximately linear up to the 20mA operating current, and almost linear up to the absolute maximum of 50mA.
Various LED indicators drawing 2mA or 1mA are available, with intensities typically ranging be- tween 1.5mcd and 2.5mcd. This low light output is still bright enough for viewing in a lab-bench environment. Low-current blue LEDs are not available. The only LEDs that draw as little as 1mA are red, as this is the most efficient type.
Using a higher value series resistor with a generic LED will of course reduce its current consumption, and some light will be visible so long as the forward voltage across the LED remains at its minimum level or above.
Forward Voltage
Red is the color that requires not only the least forward current, but the lowest forward voltage. In the range of 1.6VDC to 1.7VDC, all the LEDs are red. Typical forward voltages for various colors are shown here:
• Infrared LED: 1.6V to 2V
• Red LED: 1.6V to 2.1V
• Orange LED: 1.9V to 2.1V
• Amber LED: 2V to 2.1V
• Yellow LED: 2V to 2.4V
• Green LED: 2.4V to 3.4V
• Blue LED: 3.2V to 3.4V
• Ultraviolet LED: 3.3V to 3.7V
• White LED: 3.2V to 3.6V
Color Rendering Index
The color rendering index (CRI) evaluates the fidelity with which a light source is capable of dis- playing the full visible spectrum. It ranges from a perfect score of 100 down to 0 or even lower (sodium-vapor street lighting has a negative value). Computing the index requires standard reference color samples and has been criticized for generating scores that do not correlate well with subjective assessments.
Incandescent bulbs can have a CRI of 100, while an uncorrected white LED may score as low as 80.
Life Expectancy
Because the light output from an LED tends to decrease very gradually with time, the life expectancy is often defined as the number of hours required for the output to diminish to 70% of its output when new. Life expectancy is commonly stated on datasheets for high-brightness white LEDs, but is often omitted from datasheets for LED indicators.
Unlike incandescent lamps and fluorescent lights, LEDs do not have a shorter lifespan if they are frequently cycled on and off.
Light Output and Heat
The light intensity of an LED, measured in mcd, can vary from a few mcd to a maximum of 40,000mcd. Intensities above 30,000mcd generally are achieved by limiting the view angle to 15 degrees or less. Because the candela is weighted toward the central, green segment of the visible spectrum, green LEDs are likely to have a relatively high mcd rating. LEDs rated between 20,000mcd and 30,000mcd, with a view angle of 30 degrees, are almost all green.
Datasheets may often include a derating curve showing the lower limit that should be placed on forward current through an LED indicator when its temperature increases. In Figure 22-9, the LED should be operated only within the boundary established by the green line.
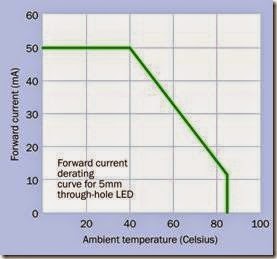
Figure 22-9. Safe operation of an LED entails limiting the forward current if the temperature increases. The green line shows the boundary for operation of this particular component.
View Angle
An LED formed from transparent epoxy or silicone (either water-clear or tinted) will create a well-defined beam with a view angle as narrow as 4 degrees or as wide as 160 degrees (in a few instances). The most common view angles for LED indicators are 30 degrees and 60 degrees.
Datasheets for LED indicators often include a spatial distribution graph showing the relative intensity of the light when viewed at various angles from the axis of the LED. The spatial distribution graph in Figure 22-10 is for an LED with a view angle defined as 40 degrees. This is the angle at which the relative luminous intensity diminishes to 50%.
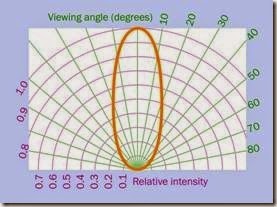
Figure 22-10. A spatial distribution graph shows the rela- tive intensity of light from an LED at various view angles.
The view angle is of special concern in devices such as flashlights, where the spread of the beam affects the functionality.
How to Use It
Like all semiconductor devices, LEDs can be impaired by excess forward current and may break down irreversibly if subjected to excess reverse voltage. Their limits for reverse voltage are much lower than those of a rectifier diode. They are also vulnerable to heat, but are not particularly vulnerable to static electricity.
Polarity
A through-hole LED will have two leads of un- equal length. The longer lead connects internally with the anode of the diode, and should be wired externally to the “more positive” side of a power source. The shorter lead connects internally with the cathode of the diode, and should be wired externally to the “more negative” side of a power source.
To remember the functions of the leads, consider that the plus sign would be twice as long as a minus sign if its horizontal and vertical stroke were disassembled and placed end to end.
If a round LED has a flange around its base, a flat spot in the flange will be closest to the cathode side of the component.
Series Resistor Value
Because the effective internal resistance of a di- ode is not a constant value at different voltages, a trial-and-error approach may be necessary to determine the ideal value for a series resistor with an LED indicator. For this purpose, a trimmer potentiometer can be used with a sample LED while measuring the current through it and the voltage drop across it. A fixed-value resistor can then be substituted. If the choice is between a resistor value that is a little too high and a value that is a little too low, the higher value resistor should be used.
An approximate value can be found using a very simple formula in which R is the resistor value, VCC is the supply voltage, VF is the forward volt- age specified for the LED, and I is the desired cur- rent:
R = (VCC – VF) / I
Normally a series resistor rated at 1/4 watt will be acceptable, and 1/8 watt may be used in 5VDC circuits. However, care should be taken with a power supply of 9V or higher. Suppose an LED is rated for 1.8V forward voltage at 20mA. In a 5V circuit, the voltage drop across the series resistor will be:
V = 5 – 1.8 = 3.2
Therefore, the resistor must dissipate 3.2V * 20mA = 64mW. This is comfortably below the 125mW rating of a 1/8 watt resistor. However, with a 9V power supply, the voltage drop across the series resistor will be:
V = 9 – 1.8 = 7.2
Now the resistor must dissipate 7.2V * 20mA = 144mW. This exceeds the 125mW limit for a 1/8 watt resistor.
LEDs in Parallel
If multiple LEDs are to be driven in parallel, and none of them has to be switched individually, it is naturally tempting to save time by using a single series resistor for all of them. In these circumstances, assess the maximum current carefully and multiply by the voltage drop imposed by each of the LEDs, to determine the wattage of a series resistor.
Linking dissimilar LEDs in parallel is not recommended, because the threshold voltage decreases with increasing temperature. The hottest LED will therefore receive the largest current, and thus become even hotter. Thermal runaway can result.
LEDs containing their own series resistors can safely be wired in parallel.
Multiple Series LEDs
A series resistor wastes current by dissipating it as heat. In an application where two or more LED indicators will be illuminated simultaneously, the LEDs can be connected in series with a lower- value resistor, and three LEDs in series may elim- inate the need for a resistor completely, depending on the voltage of the power supply. Here again a trimmer potentiometer should be used to determine an ideal value for any series resistor that may be necessary.
Comparisons with Other Light Emitters
Because LED indicators have largely replaced ne- on bulbs and miniature incandescent lamps, comparisons are of limited importance at this point. The situation regarding LED area lighting is different in that it is still competing actively with fluorescent lights and, in some instances, halogen. A list of advantages and disadvantages for high-intensity white LEDs is given in “Com- parisons” on page 223. The advantages of incan- descent lamps are listed in “Relative Advantag- es” on page 179.
Other Applications
LEDs are used in optocouplers and in solid- state relays. Usually an infrared LED is embedded inside a chip or a plastic module, and emits light through an interior channel to activate a phototransistor. This arrangement provides electrical isolation between the switching signal and the switched current.
Some sensors use an LED paired with a photo- transistor at opposite sides of a U-shaped plastic mount. A sensor of this type can monitor industrial processes or may be found inside a photo- copy machine, to detect the presence of a sheet of paper.
What Can Go Wrong
voltage of the diode. The exception to this rule is if the internal resistance of the battery is high enough to limit the current, as in the case of button-cell batteries.
Storage Issues
LEDs of different types are often indistinguishable from each other. They can also be indistinguishable from photodiodes and phototransistors. Careful storage is mandatory, and reusing LEDs that have been breadboarded may cause future problems if they are wrongly identified.
Polarity
If the leads on an LED indicator are trimmed, and if the indicator lacks a flange in which a flat spot will identify the cathode, the component is easily misused with reversed polarity. If it is connected
Excessive Forward Voltage
Like any diode, the LED has a threshold voltage in the forward direction. If this threshold is exceeded, the effective internal resistance of the LED falls very rapidly. Current rises equally rapidly, and quickly damages the component, unless it is protected by an appropriate series resistor.
Excessive Current and Heat Exceeding the recommended value for forward current, or allowing an LED to overheat, will shorten its lifetime and cause a premature dimming of light output. LEDs generally require some current limiting or regulation (most commonly with a series resistor). They should not be connected directly to a voltage source such as a battery, even if the battery voltage matches the with a component that has limited current sourcing capability (for instance, the output pin of a digital chip), the LED will probably survive this treatment. However, maximum reverse voltage is often as low as 5VDC. To minimize the risk of errors, the anode lead can be left slightly longer than the cathode lead when they are trimmed for insertion in a breadboard or perforated board.
Internal Resistors
As previously noted, it is difficult to distinguish an LED that contains its own series resistor from another LED that does not. The two types should be stored separately, and should be reused circumspectly.